Quality Control of Stainless Steel Pipe Welding
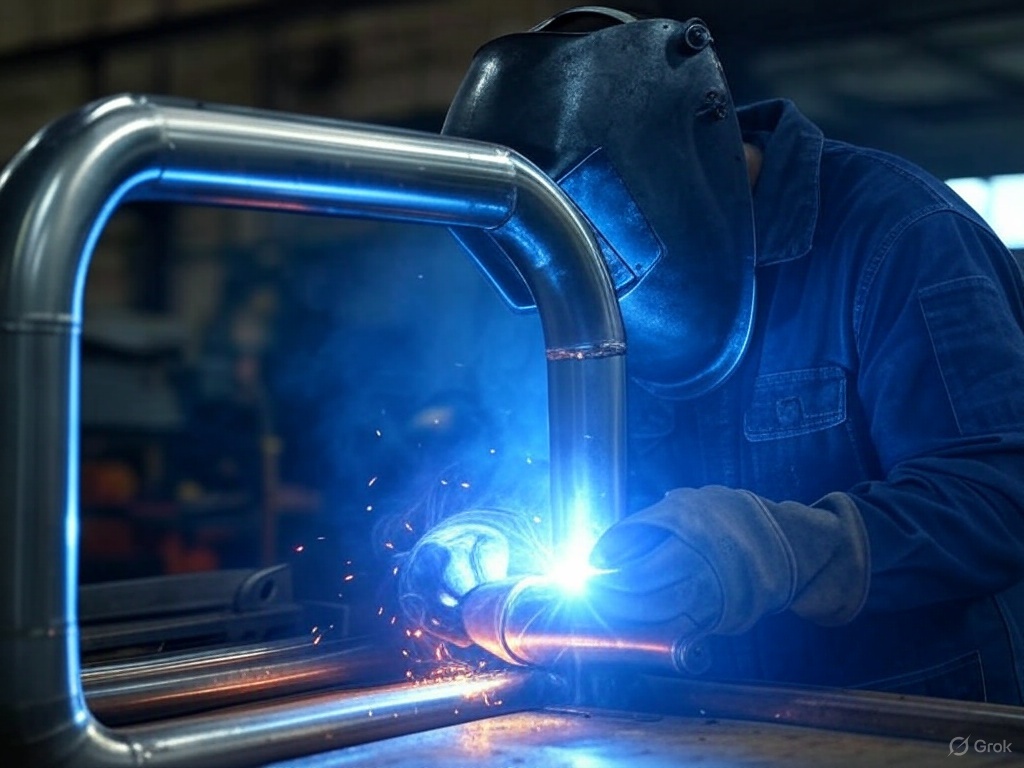
Quality Control of Stainless Steel Pipe Welding
Introduction
Stainless steel pipes are widely used in industries such as oil and gas, chemical processing, power generation, and food processing due to their excellent corrosion resistance, durability, and ability to withstand high temperatures and pressures. However, the welding of stainless steel pipes introduces unique challenges, as the material’s properties—such as low thermal conductivity, high thermal expansion, and sensitivity to contamination—make it prone to defects like cracking, distortion, and weld Imperfections. Ensuring high-quality welds is critical to maintaining the structural integrity, safety, and longevity of stainless steel piping systems.
Quality control (QC) in stainless steel pipe welding involves a systematic approach to preventing, detecting, and correcting weld Imperfections. This process encompasses material selection, welder qualification, welding procedure specification (WPS), process control, inspection, and testing. The objective is to achieve welds that meet industry standards (e.g., ASME, AWS, API) and project-specific requirements while minimizing defects and ensuring reliability. This article explores the key aspects of quality control in stainless steel pipe welding, including methodologies, tools, standards, and practical strategies, supported by tables and case studies.
Theoretical Background
Characteristics of Stainless Steel in Welding
Stainless steel is an iron-based alloy containing at least 10.5% chromium, which forms a passive oxide layer that enhances corrosion resistance. Common grades used in piping include austenitic (e.g., 304, 316), ferritic (e.g., 430), and duplex (e.g., 2205) stainless steels. Each type has distinct welding characteristics:
- Austenitic Stainless Steel: Highly weldable but prone to sensitization (chromium carbide precipitation at grain boundaries) if improperly heated, leading to intergranular corrosion.
- Ferritic Stainless Steel: Less prone to sensitization but more susceptible to grain growth and embrittlement at high temperatures.
- Duplex Stainless Steel: Offers a balance of strength and corrosion resistance but requires precise control of heat input to maintain the austenite-ferrite phase balance.
During welding, stainless steel’s low thermal conductivity causes heat to concentrate in the weld zone, increasing the risk of distortion and residual stresses. Its high thermal expansion coefficient exacerbates this issue, often resulting in warping or cracking if not properly managed.
Common Weld Imperfections in Stainless Steel Pipes
Weld Imperfections in stainless steel pipes can compromise the integrity of the system. Common defects include:
- Cracks: Hot cracking (during solidification) or cold cracking (due to hydrogen embrittlement) can occur if cooling rates or hydrogen levels are not controlled.
- Porosity: Gas entrapment in the weld pool, often due to contamination or inadequate shielding gas coverage.
- Incomplete Fusion: Failure of the weld metal to fully bond with the base material, often caused by improper welding parameters.
- Undercut: A groove at the weld toe that reduces the cross-sectional thickness, acting as a stress concentrator.
- Distortion: Warping of the pipe due to uneven heating and cooling, particularly problematic in thin-walled pipes.
Quality control aims to minimize these defects through proper material preparation, welding techniques, and inspection methods.
Quality Control Framework for Stainless Steel Pipe Welding
Pre-Welding Quality Control
Quality control begins before welding, with careful preparation to ensure optimal conditions. Key pre-welding QC measures include:
- Material Verification: Confirm that the stainless steel grade, pipe dimensions, and filler materials match the project specifications. Certificates of conformity and material test reports (MTRs) should be reviewed.
- Surface Preparation: Clean the pipe surfaces to remove contaminants like oil, grease, and oxides, which can cause porosity or cracking. Use solvents or mechanical cleaning (e.g., grinding) as needed.
- Joint Design and Fit-Up: Ensure proper joint design (e.g., V-groove, J-groove) and alignment to minimize stress concentrations and ensure full penetration. Tolerances for misalignment and gap should be within specified limits.
- Welder Qualification: Welders must be qualified per standards like ASME Section IX or AWS D1.1, demonstrating their ability to produce defect-free welds under simulated conditions.
- Welding Procedure Specification (WPS): Develop and approve a WPS detailing the welding process (e.g., TIG, MIG), parameters (e.g., current, voltage, travel speed), filler material, shielding gas, and preheat requirements.
Welding Process Control
During welding, strict control of the process is essential to produce high-quality welds. Key process control measures include:
- Shielding Gas Selection: Use high-purity inert gases (e.g., argon or argon-helium mixtures) to prevent oxidation of the weld pool. Ensure proper gas flow rates and coverage, including back-purging for root passes in austenitic stainless steel.
- Heat Input Control: Maintain heat input within specified limits to avoid sensitization or excessive grain growth. Heat input (in kJ/mm) can be calculated as:
Heat Input = (Voltage * Current * 60) / (Travel Speed * 1000)
Typical heat input for austenitic stainless steel should be kept below 1.5 kJ/mm to prevent sensitization.
- Interpass Temperature Control: Limit interpass temperature (e.g., 150°C for austenitic stainless steel) to prevent overheating and reduce residual stresses.
- Filler Material Selection: Use filler metals with matching or slightly over-alloyed compositions (e.g., 316L filler for 316 stainless steel) to ensure corrosion resistance and mechanical properties.
- Monitoring Welding Parameters: Continuously monitor and record voltage, current, travel speed, and gas flow to ensure compliance with the WPS.
Post-Welding Quality Control
After welding, inspection and testing are performed to verify weld quality. Key post-welding QC measures include:
- Visual Inspection: Check for surface defects like cracks, undercut, or incomplete fusion using magnifying tools or borescopes for internal welds.
- Non-Destructive Testing (NDT): Employ methods such as radiography (RT), ultrasonic testing (UT), dye penetrant testing (PT), or magnetic particle testing (MT) to detect subsurface defects.
- Destructive Testing: Conduct tests like tensile testing, bend testing, or impact testing on sample welds to evaluate mechanical properties.
- Post-Weld Heat Treatment (PWHT): Apply PWHT if required (e.g., for stress relief in heavy sections) while ensuring temperature and cooling rates do not induce sensitization.
- Documentation: Maintain detailed records of welding parameters, inspection results, and test reports for traceability and compliance.
Standards and Specifications for Quality Control
Several industry standards provide guidelines for quality control in stainless steel pipe welding. Key standards include:
- ASME B31.3: Process Piping, specifies requirements for welding and inspection in chemical and petrochemical plants.
- AWS D1.6: Structural Welding Code—Stainless Steel, covers welding and qualification procedures for structural applications.
- API 1104: Welding of Pipelines and Related Facilities, provides guidelines for pipeline welding, including stainless steel.
- ISO 3834: Quality Requirements for Fusion Welding of Metallic Materials, outlines general QC principles.
These standards specify acceptance criteria for weld Imperfections, such as maximum allowable sizes for porosity, cracks, and undercut, ensuring welds meet safety and performance requirements.
Common Weld Imperfections and Control Measures
Table 1 summarizes common weld Imperfections in stainless steel pipe welding, their causes, and quality control measures to mitigate them.
Defect | Causes | Control Measures |
---|---|---|
Cracks | High heat input, rapid cooling, hydrogen contamination | Control heat input, use low-hydrogen filler materials, preheat if necessary |
Porosity | Contaminated surfaces, inadequate shielding gas | Clean surfaces thoroughly, ensure proper gas flow and coverage |
Incomplete Fusion | Low heat input, improper welding technique | Adjust welding parameters, train welders on proper techniques |
Undercut | Excessive current, improper electrode angle | Optimize current settings, maintain correct electrode angle |
Distortion | Uneven heating and cooling | Use tack welds, control heat input, apply clamping or fixtures |
Inspection and Testing Techniques
Non-Destructive Testing (NDT)
NDT methods are critical for detecting weld Imperfections without damaging the pipe. Common NDT techniques for stainless steel pipe welding include:
- Radiographic Testing (RT): Uses X-rays or gamma rays to detect internal defects like porosity and inclusions. Suitable for thick-walled pipes but requires safety precautions.
- Ultrasonic Testing (UT): Uses high-frequency sound waves to detect subsurface defects. Effective for detecting cracks and lack of fusion.
- Dye Penetrant Testing (PT): Applies a dye to the weld surface to reveal surface-breaking defects like cracks and porosity. Ideal for austenitic stainless steel.
- Magnetic Particle Testing (MT): Detects surface and near-surface defects in ferritic stainless steel using magnetic fields and iron particles.
Table 2 summarizes the applicability of NDT methods for stainless steel pipe welding.
NDT Method | Defects Detected | Applicability | Limitations |
---|---|---|---|
Radiographic Testing (RT) | Porosity, inclusions, cracks | Thick-walled pipes | High cost, radiation hazards |
Ultrasonic Testing (UT) | Cracks, lack of fusion | All thicknesses | Requires skilled operators |
Dye Penetrant Testing (PT) | Surface cracks, porosity | Austenitic stainless steel | Limited to surface defects |
Magnetic Particle Testing (MT) | Surface cracks, near-surface defects | Ferritic stainless steel | Not applicable to austenitic grades |
Destructive Testing
Destructive testing involves sacrificing a sample weld to evaluate its mechanical properties. Common tests include:
- Tensile Testing: Measures the weld’s strength and ductility by pulling it apart until failure.
- Bend Testing: Assesses ductility and fusion by bending the weld sample to a specified angle without cracking.
- Impact Testing: Evaluates toughness by striking the weld with a pendulum to measure energy absorption.
These tests are typically performed on test coupons prepared during welder qualification or production welds.
Practical Quality Control Strategies
Training and Certification
Welders and inspectors must be adequately trained and certified to ensure consistent quality. Training programs should cover stainless steel metallurgy, welding techniques, and defect identification. Certification per ASME Section IX or AWS standards ensures that personnel meet minimum competency requirements.
Process Monitoring and Automation
Automated welding systems, such as orbital TIG welding, can improve consistency by controlling parameters like travel speed, heat input, and gas flow. Real-time monitoring systems can record welding parameters and alert operators to deviations from the WPS.
Environmental Control
Welding should be performed in a controlled environment to minimize contamination. Use clean rooms or wind barriers to protect the weld area from dust, moisture, and drafts, which can affect shielding gas coverage and weld quality.
Case Studies
Case Study 1: Oil Refinery Piping System
In a recent oil refinery project, 316L stainless steel pipes (4-inch diameter, 6 mm wall thickness) were used for a process piping system. Initial welds showed porosity and cracking due to inadequate back-purging and high heat input. Quality control measures were implemented, including:
- Using 99.99% pure argon for back-purging.
- Reducing heat input to 1.2 kJ/mm.
- Conducting 100% RT on all welds.
After these adjustments, defect rates dropped from 15% to 2%, meeting ASME B31.3 acceptance criteria.
Case Study 2: Food Processing Plant
A food processing plant installed 304 stainless steel pipes (2-inch diameter, 3 mm wall thickness) for a sanitary system. Weld Imperfections like undercut and lack of fusion were detected during visual inspection. The QC team implemented:
- Training welders on proper TIG welding techniques.
- Using dye penetrant testing for all welds.
- Ensuring interpass temperature did not exceed 120°C.
These measures reduced Imperfections to below 1%, ensuring compliance with sanitary standards.
Conclusion
Quality control in stainless steel pipe welding is a multifaceted process that requires attention to material properties, welding techniques, and inspection methods. By implementing rigorous pre-welding, in-process, and post-welding QC measures, weld Imperfections such as cracks, porosity, and distortion can be minimized. Standards like ASME B31.3 and AWS D1.6 provide a framework for ensuring weld quality, while NDT and destructive testing offer reliable methods for defect detection and validation.